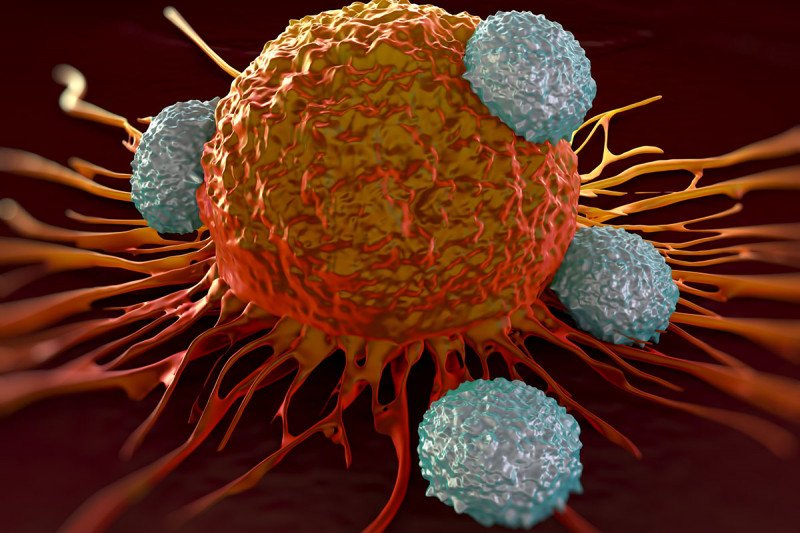
New and emerging information about chimeric antigen receptor (CAR) T cell therapy in the setting of hematologic malignancies is informing strategies for overcoming obstacles in solid tumors.
In our paper, published recently in the journal Expert Review of Hematology, we discuss the challenges with current CD19-targeted CARs, obstacles to adopting CAR T cell therapy in solid tumors, and various strategies for addressing these issues. (1)
This CAR T Update Part 2 summarizes the unique challenges with using CARS to defeat solid tumors, and how learning from preclinical and clinical research in hematologic malignancies is informing new strategies and research efforts. We discuss antigen selection, CAR design, trafficking CAR T cells to tumor sites, and overcoming challenges associated with an inhibitory tumor microenvironment (TME), T cell exhaustion, and T cell proliferation. We also touch on issues related to production logistics and financial accessibility.
Refer to CAR T Update Part 1 to read about learning and research avenues under investigation for CAR T cell therapy in hematologic malignancies. In Part 1, we cover the main issues of antigen escape, T cell persistence, and safety, and the breadth of strategies under investigation to address them.
Scientists at Memorial Sloan Kettering Cancer Center (MSK) pioneered CAR T cell therapy as a cancer treatment and were the first to demonstrate that CD19 was a robust target in the setting of hematologic cancers. Today, we continue to investigate how to improve the efficacy of CAR T cells and extend their use to solid tumors, including breast cancer, ovarian cancer, lung cancer, and mesothelioma.
Antigen selection
An ideal target antigen has a high and universal expression on tumor cells, no or low expression on normal cells, and indispensable to the tumor. CD19 is a unique, optimal target since its expression is limited to B-cells at any stage of differentiation. On-target, off-tumor toxicity is limited to normal B cells, and the side effect of hypogammaglobulinemia can be easily managed with immunoglobulin infusions. (2)
Finding another antigen with these optimal characteristics has been challenging, in part because most CARs to date have targeted cell surface molecules. Advances in RNA sequencing, microarray analysis, and proteomics have made it possible to identify new preclinical targets, such as the recent discovery of glypican2 (GPC2) in neuroblastoma, (3), (4) and other possibilities for combinations in acute myeloid leukemia (AML). (5)
Combining two tumor antigens and either scFv to activate the CARs can help overcome heterogeneous antigen expression as well as the presence of stromal and fibrous tissue in solid tumors. Incorporating a conditional activation switch that requires both tumor antigens to be present but not the normal antigen can improve safety. (6), (7), (8)
Another strategy involves manipulating the TME from an immunosuppressive to the pro-inflammatory state by using CARs that deliver cytokines locally to the TME (9), (10) or by another process known as antigen spreading. (11), (12) By Antigen Spread, the CAR T cells work to reveal previously unavailable neo-antigens that are presented to endogenous cytotoxic T cells, which propagate and continue to attack the tumor on their own.
Tumor stromal and vascular tissues can also be targeted, using the antigen as a homing beacon to direct the CAR T cells to the TME, where they deliver cytokines to aid tumor kill with the help of locally infiltrated immune cells. (13), (14), (15)
CAR Design
Cell engineers can optimize CAR design based on the target antigen and the scFv. Spatial orientation on first engagement with an antigen can drive or impede the cytotoxic effects of CARs. (16) Further, it may also be necessary to individualize CAR design based on the target antigen. (17) Examples of optimizing orientation are the CD22-directed CAR T cells for treating B-ALL (18) and prostate stem cell antigen (PSCA)-targeted CARs. (19)
For tyrosine-protein kinase transmembrane receptor (ROR1)-specific CARs used to target epithelial malignancies such as ovarian, breast, and lung cancers, (20) the affinity and length of the extracellular spacer domain determined the lead scFv candidate with the best potency. (21) Mutating binding sites on the extracellular spacer domain helps avoid transgenic immune responses and CAR T cell rejection. (19)
Trafficking CAR T Cells to the Tumor Site
Chemokine receptors can help systemically administered CARs home to the tumor site. However, this strategy is challenging because tumors secrete different types of chemokines. One preclinical model showed that chemokine receptor type 2b (CCR2b) expression on GD2-CAR T cells improved homing to neuroblastoma tumor cells (22) and pleural mesotheliomas. (23) Similarly, blocking protein kinase A in T cells from reaching the immunological synapse improved T cells’ ability to infiltrate tumors with increased expression of CXC motif chemokine receptor 3 (CXCR3) on T cells. (24) Clinical trial NCT03602157 is currently testing this strategy.
An entirely different approach is to deliver CAR T cells directly into the tumor or in close proximity. This approach may be particularly useful with central nervous system (CNS) tumors since systemically delivered CARs may not efficiently cross the blood-brain barrier. Some promising work has been conducted using direct delivery approach using CARS directed against IL-13Ralpha2 antigens overexpressed in some brain tumors, (25), (26) and against MUC-16 (ecto) antigen for ovarian cancer (27) and this approach is safe. (28) Several trials underway include NCT02414269, NCT02498912, NCT03818165, NCT03585764, NCT03500991, NCT02442297, NCT03638167, NCT03696030, and NCT03907527.
Oncolytic viruses can infect and lyse tumor cells. They can release neoantigens that encourage antitumor response and can be armed with cytokines to enhance efficacy. (29), (30) Studies to map T cell trafficking using indium labeling (31) or novel imaging methods (32) are invaluable for future learning. One active trial of note, tracking infused CAR T cells is NCT03283631 (enrollment temporarily suspended until a protocol amendment is approved).
Hostile TME, T cell Exhaustion, and Proliferation
The TME may be immunosuppressive to CAR T cell therapies and, the stroma and the extracellular matrix can prevent effective migration to cancer cells. A hostile TME may be caused by: the presence of inhibitory cells such as T-regulatory cells, tumor-associated macrophages, or myeloid-derived suppressor cells (MSDCs); programmed death-ligand 1 (PD-L1) expression on tumor cells; metabolic competition; and, chronic T cell receptor signaling that leads to T cell exhaustion. (33)
Scientists are using several different strategies to overcome these challenges. CAR T cells engineered to produce heparanase have demonstrated improved penetration of CARS within tumors by degrading the extracellular matrix. (34) There has also been considerable research lately related to cancer-associated fibroblasts, which play a role in extracellular matrix remodeling. (35), (36)
“Armored” CARS, engineered to secrete scFvs or cytokines in the TME, have improved the efficacy of CAR T cell therapy. (37) IL12-secretion can skew the TME to a pro-inflammatory state, inhibit T-regs, decrease the presence of TAMs, and resist PD-L1-mediated inhibition, helping CARs persist and boosting their cytotoxicity. (27), (38), (39), (40) IL-18 secreting CAR T cells have increased proliferation and enhanced antitumor efficacy compared to second-generation approaches (41), (42) resulting in a pro-inflammatory tumor microenvironment. (43)
There are at least four active “armored” CAR T cell trials in the United States: NCT02498912, NCT03721068, NCT03635632, and NCT03085173. In the latter study, led by MSK researchers, patients with relapsed/refractory non-Hodgkin lymphoma or chronic lymphocytic leukemia are being treated with 19-28z/4-1BBL armored CAR T cells. Early data showed that sixteen of 28 patients achieved a complete response, which was sustained at a median follow-up of 169 days in eight patients. (44)
T4 immunotherapy is an exciting approach that involves using CAR T cells directed against various antigens and that co-express a chimeric cytokine receptor. (45) Fusing the IL4 receptor ectodomain to a beta domain, the signaling moiety for IL2 and IL15 takes advantage of the abundance in the TME of the immunosuppressive cytokine IL4 while also transforming the signal into a T-cell stimulatory signal, mimicking IL2 and IL15 cytokine activation required for T cell growth and persistence.
Combining checkpoint blockade with CAR T cell technology is another area of significant research effort. Strategies include systemic administration of checkpoint blocking antibodies, (46); using PD-1-blocking-scFV-secreting CAR T cells; (47) and editing out PD-1/LAG-3 and other inhibitory genetic loci on T cells with CRISPR-Cas9 before modifying them to express the CAR. (48), (49), (50) There are several trials underway testing these above approaches: NCT01818323, NCT04003649, NCT02414269, NCT03726515, NCT03747965, and NCT03525782. The NCT02414269 trial, led by MSK researchers, is testing intrapleural mesothelin-directed CAR T cells. The subset of patients who received an anti-PD-1 agent (51) has shown the best overall response so far, consistent with preclinical research. (52)
Metabolic competition for oxygen and nutrients in the TME is fierce, so using targeted drugs to deplete indoleamine 2,3-dioxygenase (IDO) (53) or inhibit glycolysis (54) are methods of interest to improve the efficacy of CAR T cell therapy. Another strategy is to engineer T cells to express RAS homolog enriched in brain (RHEB) to enable activation of mammalian target of rapamycin (mTOR), resulting in increased glycolysis and expansion of T cells. (5)
Scientists are also investigating ways to overcome the issue of exhaustion of CAR T cells in the TME. One method is to temporarily cease zeta phosphorylation in T cell receptors with small molecules that help regulate CAR expression levels and restore functionality. (56) Another technique involves eliminating redundancy in CAR signaling by eliminating extra immunoreceptor tyrosine-based activation (ITAM) motifs in the CD3zeta signaling domain. (57)
Production Logistics and Financial Accessibility Issues
From the initial concept of CAR T cell therapy as an immunotherapy strategy, to effortlessly genetically modifying T cells, we have reached a stage where mass production to meet clinical needs is possible. This progress has been accomplished rapidly — in under 25 years.
However, large-scale production requires addressing manufacturing inadequacies, the financial cost, and allo-incompatibility of the current FDA-approved products.
Strategies that yield superior products are using different cytokines for better expansion (58) and elutriation, (59) which involves separating lymphocytes from monocytes and granulocytes. Alkylators and anthracycline chemotherapies, which are cornerstone agents in several solid tumors, can lower the quality of T cell products. (60) A strategy to improve yield is to identify high-risk patients and harvest and store their leucocytes in advance, minimizing their exposure. However, this approach may be cost-prohibitive, since not every patient at high risk for relapse will require frozen cells modified into CARs.
Off-the-shelf CAR T cell products can shorten production time, costs, and reach a broader patient population. Recommended strategies to generate these products are using gene-editing technologies such as CRISPR-Cas9/TALEN to remove endogenous T cell receptors, (48), (61) or using natural killer cells (NCT02742727) or cord blood-derived cells (NCT03881774).
Costs associated with producing CAR T cells remain incredibly high, at about $370,000 per patient. (62) Allogeneic CAR T cells, increased production scalability, and distant domestic and offshore production may help improve financial accessibility. (63)
The paper was not funded. Dr. Namuduri is an adhoc consultant for Cellectar Biosciences. Dr. Brentjens receives royalties and grant support from JUNO Therapeutics and is a consultant for JUNO/Celgene. The authors have no other relevant affiliations or financial involvement with any organization or entity with a financial interest in or financial conflict with the subject matter or materials discussed in the manuscript apart from those disclosed.