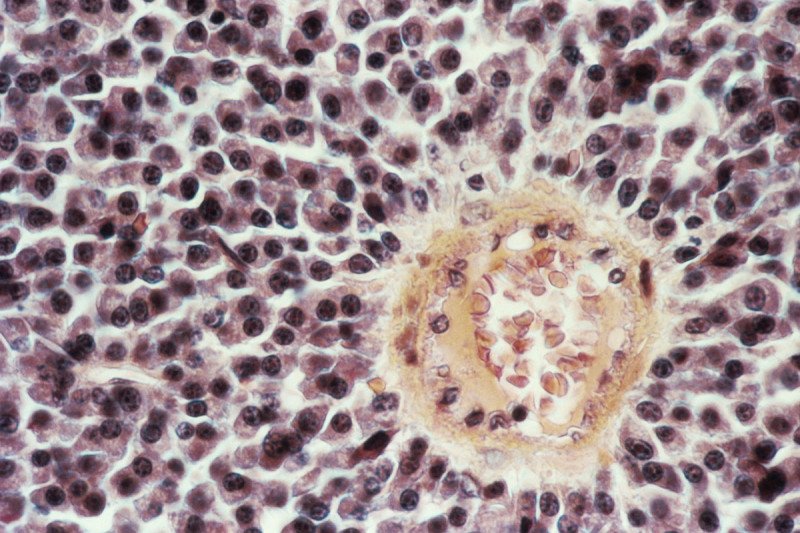
A single surviving myeloma cell can seed accelerated relapse, and more than 20 percent of nonsynonymous mutations at relapse may be induced by exposure to chemotherapy such as platinum and high-dose melphalan after autologous stem cell transplant, according to our research, published recently in Nature Communications.
Our study is the first to investigate by whole genome sequencing the anatomical evolutionary process of multiple myeloma relapse in a systematic fashion. Previously, it has been unclear whether new disease sites at relapse reflect pre-existing, undetected disease localization, or new dissemination of disease seeding.
We interrogated mutational signatures in multiple concurrent samples obtained at warm autopsy, in patients with relapsed/refractory multiple myeloma. Our results show that an evolutionary process, during which distinct clones harboring unique drivers are selected and expanded at various anatomic sites, promotes multiple myeloma seeding. Using chemotherapy-related mutational signatures as a genomic barcode linked to a known timepoint in each patient’s life, we demonstrated that relapse can be driven by a single surviving cell that may disseminate throughout the body in a very short time frame. The spatio-temporal patterns of relapse were strikingly different from those observed during spontaneous evolution before diagnosis and initial exposure to therapy. (1)
Accelerated anatomic dissemination is likely the result of a combination of the selection of more aggressive/proliferative clones and treatment-related immunosuppression. The first factor is typically unpredictable and undetectable with current bulk sequencing technologies. However, strategies promoting immune reconstitution may prevent acceleration and help reduce the incidence of relapse. (1)
Our findings highlight the importance of considering the complex spatial and temporal heterogeneity of multiple myeloma when evaluating treatment-response and minimal residual disease. It also provides a strong rationale for comprehensive genomic characterization to enhance clinical decision making.
Multiple Myeloma Mutational Processes
Multiple myeloma is characterized by a long and complex evolutionary process through two clinically defined precursor stages: monoclonal gammopathy of uncertain significance and smoldering multiple myeloma. (2), (3), (4), (5) Disease progression involves branching evolutionary patterns and clonal sweeps together with local evolution and expansion of cancer cells in various anatomical sites. (6), (7) A range of clones compete for dominance and survival in local environments, leading to increased genomic heterogeneity at distinct sites. (8), (9), (10), (11), (12), (13) Similar evolutionary patterns have also been observed in patients with relapsed multiple myeloma. (14), (15), (16)
Different processes cause somatic mutations in cancer genomes, and each generates a unique mutational signature. Researchers have described more than 40 mutational signatures, by considering each single nucleotide variant (SNV) together with its neighboring bases at 5’ and 3’. Some of these mutations are associated with defective DNA repair mechanisms, exposure to exogenous carcinogens, or radical oxygen stress. (17), (18)
We recently described the landscape of active mutational processes in multiple myeloma. At diagnosis, the disease is shaped by seven main mutational processes, including five with a recognized etiology: activation-induced cytidine deaminase (AID; SBS9), aging (SBS1 and SBS5), and apolipoprotein B mRNA Editing Catalytic Polypeptide-like (APOBEC; SBS2 and SBS13). (19), (20), (21), (22), (23)
At relapse, we discovered a new mutational signature named SBS-MM1, which is associated with exposure to the alkylating agent melphalan, (22) used for treating multiple myeloma and for conditioning before autologous stem cell transplant. Similar to chemotherapy-related mutational signatures in other cancers, each surviving myeloma cell exposed to melphalan acquires a unique set of mutations detectable by bulk sequencing — only if an exposed cell is positively selected and expands. (18), (24), (25) Therefore, two different multiple myeloma localizations after melphalan exposure may result from three different scenarios: (1) all cancer cells in both areas share identical melphalan-related mutations, suggesting that a single cell survived melphalan exposure and seeded both; (2) each localization has a unique set of melphalan-related mutations, suggesting that cells in these areas pre-existed exposure; or, (3) myeloma cells may have been reintroduced during autologous stem cell transplant, avoiding melphalan exposure.
Study Design
We looked for mutational signatures in whole-genome sequences of 25 tissue samples collected at warm autopsy from four patients with relapsed/refractory multiple myeloma, including 21 tumor and four non-tumor samples taken from uninvolved skeletal muscle. We also interrogated an additional set of 125 whole exomes from 51 patients. (14) The multiple concurrent samples were obtained from rarely biopsied anatomical sites from patients who had consented to autopsy and sample collection.
To explore the evolution of multiple myeloma at relapse, we reconstructed the clonal and subclonal composition of each patient in the whole-genome and whole-exome sequencing cohorts by creating phylogenetic trees. Mutations shared as clonal by all samples from the same individual formed the tree trunk, and later clonal, or subclonal clusters formed the branches. (22), (26)
Study Findings
Phylogenetic Trees and Disease Seeding
Whole-genome sequencing identified a median of 10,938 mutations (range 6,977 to 13,239). The phylogenetic tree trunk of the two longer-surviving (greater than 7 years from diagnosis) patients in the whole-genome sequencing cohort accounted for 80.5 and 69 percent of all mutations. In contrast, the trunk for two patients with a shorter survival (approximately 3 years) was shorter and had significantly fewer mutations, representing 34 and 22 percent of the total mutation burden. (1)
Interestingly, each disease site in the whole-genome sequencing cohort had a unique set of genomic drivers and aberrations, reflecting distinct evolutionary processes and subclonal selection and expansion. The majority of these aberrations were single and complex structural variants and copy number aberrations, confirming their essential role in multiple myeloma progression and evolution. (15), (27), (28)
All patients had at least one clone with a mutation associated with the RAS pathway. Mutations in known driver genes (21) were rarely identified in branches of the phylogenetic tree, in contrast to newly diagnosed multiple myeloma. (1)
Across both whole-genome and whole-exome sequencing cohorts, we observed a median of 57-nonsynonymous SNVs per patient (range 21 to 464). Interestingly, patients with relapsed disease showed a higher number of nonsynonymous SNVs versus baseline in both cohorts. This finding was independent of the number of subclones and the coverage. (1)
Mutational Signatures Landscape
We defined the mutational signature profile for each sample according to our recently published workflow. (19) In addition to eight known mutational signatures, (19), (21), (22) we extracted a new signature associated with melphalan exposure called SBS-MM1. (1) It is similar to the recently reported SBS35, which has been associated with exposure to platinum-based chemotherapy. (18), (24), (25), (29)
SBS-MM1 and its transcriptional strand bias were observed in all patients in the whole-genome sequencing cohort, consistent with our previous report. (27) SBS35 was identified only in two patients who had received platinum-based chemotherapy, as expected. In the whole-exome sequencing cohort, both SBS-MM1 and SBS35 mutational signatures were observed only with mutations acquired or selected after treatment. These observations suggest that the exposure to distinct chemotherapies influence the mutational landscape of relapsed multiple myeloma. (1)
Next, we investigated the impact of chemotherapy-related mutations on genomic profiles across both cohorts and estimated the contribution of SBS35 and SBS-MM1 among nonsynonymous SNVs. Interestingly, 26 percent (95% CI: 20–32 percent) of all nonsynonymous mutations at relapse were caused by one of these two mutations, adding further evidence that exposure to chemotherapy plays a role in increasing genomic complexity and more aggressive disease at relapse. (1)
We reconstructed the timeline of mutational processes for each patient. SBS9 was detected mostly in the trunk of the phylogenetic trees, and APOBEC activity was detected in both clonal and subclonal clusters. (5), (9), (21), (22) SBS35 was only discovered in the branches of two patients who relapsed after platinum-based therapy. SBS-MM1 demonstrated a heterogeneous landscape. For example, in one patient’s tree, we identified it in the trunk and all first-level branches, but not in any second- or third-level branches. This profile was consistent with a melphalan signature common to all cells, with another subset of melphalan-induced mutations that resulted from a second exposure. In a second case, all SBS-MM1 mutations were in the trunk, consistent with exposure to high-dose melphalan followed by autologous stem cell transplant received in front-line therapy. Finally, we found SBS-MM1 in the trunk and both SBS35 and SBS-MM1 in all the latest branches of the tree, reflecting front-line high-dose melphalan followed by autologous stem cell transplant, as well as salvage platinum-based chemotherapy followed by allogeneic stem cell transplant with melphalan conditioning used at clinical relapse. (1)
For these three patients, the large number of SBS-MM1-related mutations shared by all of the different sites could only be explained by the existence of a recent common ancestor (i.e. a single cell) selected after high-dose melphalan therapy followed by autologous stem cell transplant. Further, differences between anatomic sites were not associated with pre-existing unidentified subclones, but by dissemination from a single propagating cell that survived high-dose melphalan followed by autologous stem cell transplant.
In one patient, the integration of clinical, radiological and genomic data suggested that a single propagating myeloma cell survived exposure to high-dose melphalan and drove relapse in less than 25 months. (1) This temporal pattern suggested that systemic seeding of a single clonal cell can occur rapidly at relapse. This was confirmed by exploring differences between whole exome sequencing data obtained from multiple myeloma samples collected from different anatomic loci from both newly diagnosed and relapsed multiple myeloma patients (n=125 from 51 patients) that were previously published. (14)
Advancing Multiple Myeloma Cancer Research
At MSK, our multidisciplinary experts collaborate to discover insights that advance the understanding of the underlying biology of cancer. Insights may lead to new targets and treatment approaches that have the potential to improve patient outcomes.
The present study represents a collaboration of researchers and clinicians from various departments at MSK: the Adult Bone Marrow Transplant Service, the Myeloma Service, and the Center for Hematological Malignancies, Department of Medicine; the Human Oncology & Pathogenesis Program; the Cytogenetics Laboratory and Hematopathology Service, Department of Pathology; the Department of Radiology; and, the Department of Epidemiology and Biostatistics.
We are currently conducting 26 clinical trials testing innovative treatment approaches for multiple myeloma, including chimeric antigen replacement (CAR) T cell therapies, immunotherapies, personalized high dose conditioning regimens prior to transplant, and novel chemotherapies for patients with recurrent or persistent disease.
Sequence data that support the findings of this study have been deposited in the European Genome-phenome archive.
This research was supported by the Memorial Sloan Kettering Cancer Center NCI Core Grant (P30 CA 008748) and the Susan and Peter Solomon Divisional Genomics Program. Dr. Maura is supported by the American Society of Hematology, the International Myeloma Foundation, and The Society of Memorial Sloan Kettering Cancer Center.
The authors of this Clinical Update & Insights article declare no competing interests. For disclosures from other authors on the study, please refer to the paper.