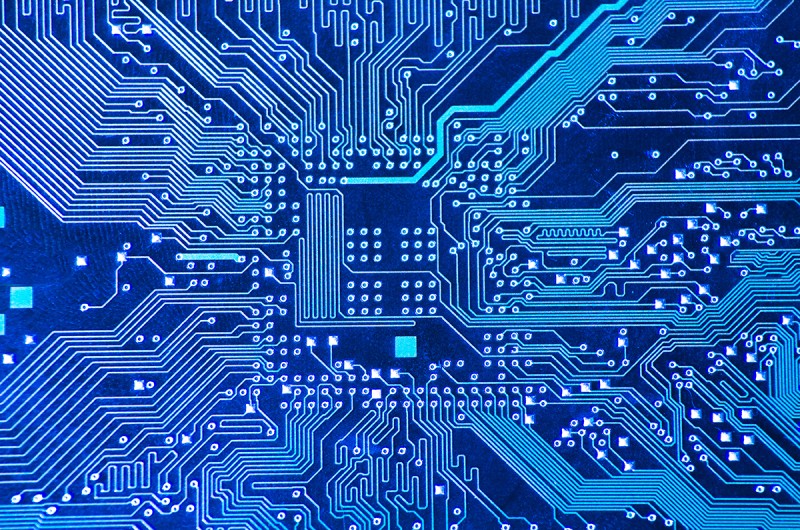
A mazelike circuit of metabolic pathways underlies all cell behavior. Glitches in these pathways can fuel cancer.
When Watson and Crick solved the structure of DNA in 1953, they launched an intellectual gold rush in biology. Suddenly, everyone wanted to study DNA, and a field was born — molecular biology — devoted to understanding how DNA serves as a blueprint for life.
What got left behind in this mad scramble was the study of metabolism, the mazelike circuit of biochemical reactions that allow living things to, for example, extract energy from food and make new cell membranes.
But metabolism is making a comeback. Researchers are once more looking to this complex network of chemical reactions for answers about cells, including what goes awry in cancer.
“The time has come for cancer biologists to dust off their biochemistry textbooks,” Craig Thompson, Memorial Sloan Kettering’s President and CEO, wrote in an influential 2006 article. “It seems there are a few chapters that still need to be written.”
Dr. Thompson has been at the vanguard of this return to metabolism. His lab has made several key discoveries that have fundamentally changed how we think about cancer. He’s also served as one of the field’s greatest champions, encouraging others to give metabolism a second look.
A Missing Link
The recent discovery that touched off the greatest excitement was the finding that the products of metabolism — the various chemicals that get spun off when nutrients are broken down — can themselves influence which genes are turned on or off in a cell. Metabolic products, in a sense, form a missing link between what a cell is eating and its genetic program. This makes sense, biologically speaking.
“If you’re a cell and you’re going to turn on genes, you want to know you have the resources to do that,” Dr. Thompson says. This is true whether the cell is planning to divide or perform some other labor-intensive task, like make antibodies or secrete milk.
Back in 2009, he and his colleagues proposed that the way cells make this link is through what scientists now call epigenetic mechanisms. That is, cells use metabolic products to modify DNA — not by changing the sequence of As, Cs, Gs, and Ts, but by altering how accessible the DNA is to the gene expression machinery.
“This was a transformative idea,” says Ming Li, an immunologist at the Sloan Kettering Institute who studies metabolism in immune cells. “The metabolic pathways communicate with cell signaling pathways, including the gene expression program, through epigenetic mechanisms.”
Targeting Metabolism
There are direct therapeutic implications of the discovery that metabolism affects gene expression. For example, some cancers, including certain leukemias and brain cancers, have mutations in a metabolic enzyme called isocitrate dehydrogenase (IDH). The mutated enzyme does not do its normal job and instead acquires a new function: creating a cancer-promoting molecule called 2-hydroxyglutarate (2HG).
How this molecule promoted cancer was not clear until 2012, when Dr. Thompson and others discovered that 2HG inhibits an epigenetic process called histone demethylation. (Histones are proteins around which DNA is spooled in a chromosome; methylation is adding methyl groups to molecules.) By blocking removal of methyl groups from histones, 2HG prevents cells from expressing genes important for their differentiation into mature cell types. As a result, these younger, more stem cell–like cells divide without knowing when to stop.
Drugs targeting 2HG are now in clinical testing. A trial of one drug, called AG-221, for people with acute myeloid leukemia (AML) is being led at MSK by hematologist-oncologist Eytan Stein.
What’s interesting about this drug, Dr. Stein says, is that it’s not actually killing cancer cells. “Most of our drugs for AML are cytotoxic, so patients get this prolonged period of bone marrow suppression,” he notes. “This is a drug where you don’t see that. Instead, you see the differentiation of cells into normal, healthy adult cells.”
It’s also an oral medication that patients can take as an outpatient, he says. Based on the promising results obtained thus far, the drug will be sent to the FDA before the end of the year for consideration of accelerated approval.
Warburg Redux
As exciting as recent discoveries have been, the knowledge that cancer cells have metabolic derangements is not new. Nearly a century ago, a German scientist named Otto Warburg made an interesting observation about cancer cells: They tend to suck up a lot of glucose. What’s more, they don’t process this sugar in the way that most cells do — that is, by “burning” it in mitochondria. Instead, they do what many single-celled organisms do: They ferment it, breaking down glucose into a molecule called lactate.
Dr. Warburg assumed that cancer cells must have a defect in their mitochondria, because otherwise why would they resort to this inefficient form of metabolism? He further believed this defect was the fundamental cause of cancer.
Researchers now know that this is wrong. Studies and analyses over the past decade have shown that cancer cells have working mitochondria and can perform the standard form of metabolism under some circumstances.
This raised the question of what purpose Warburg metabolism serves in cancer cells. Dr. Thompson’s proposal, which he first laid out in 2008, is that Warburg metabolism provides the necessary building blocks for cancer cells to divide uncontrollably. This idea runs counter to a century of work on cancer metabolism, but it’s proving to be a productive framework for research.
What causes metabolism to go haywire in the first place? An important clue came in 2004, when Dr. Thompson discovered that mutations in a gene called AKT — which is commonly mutated in cancer — allows cells to take up glucose without restraint. This suggested to Dr. Thompson that what cancer-causing oncogenes do, fundamentally, is alter metabolism in ways that set cells down the path to cancer.
All About T Cells
Dr. Thompson’s interest in metabolism goes back to the start of his career, when he was studying how immune cells called T cells are activated. In 1989, he showed that a molecule called CD28 was a kind of “gas pedal” for T cells, and that the gas it provided to the cell was glucose.
The idea that cells undergoing rapid division, as activated T cells do, might need access to abundant glucose might not seem an especially novel idea. But what Dr. Thompson did was show how this provision of glucose was provided by a signal from a specific protein: CD28. In that sense, CD28 acted not unlike the receptor for the hormone insulin, which signals many cells in the body to take up nutrients.
What this work has done is draw researchers back to the subject of metabolism, by showing that metabolism is crucial for everything a cell would want to do.
“That’s what Craig uniquely contributed to the field of metabolism,” Dr. Li says. “He emphasized that we need to go back to metabolism and integrate it with cellular physiology and pathology.”
“We forgot about that for a long time,” he adds.