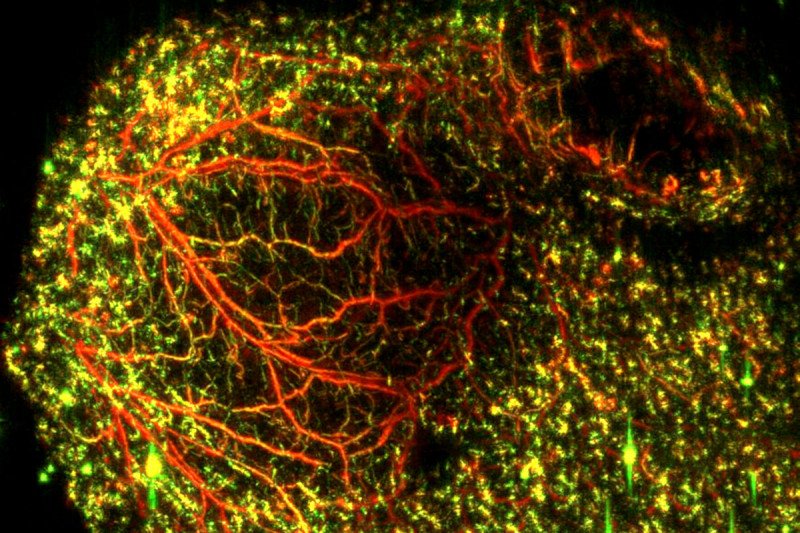
A new imaging approach uses lasers and ultrasound to generate a clear picture of blood vessels both large (red) and small (green) in mouse colon cancer tumors. The technique could be a valuable research tool to monitor how well certain drugs are working. (Katja Haedicke, Jan Grimm Lab)
Cancer needs a steady supply of nutrients to grow. Tumors ensure they are well-fed by sending out signals to trigger the formation of blood vessels that will provide their food supply, a process called angiogenesis. Over the past few decades, an important strategy has been to block angiogenesis and choke off a tumor’s blood supply.
While antiangiogenic drugs have shown some promise, their effectiveness is still limited. It can be hard to tell whether vessels are developing resistance or the drugs are being delivered adequately to the target. Researchers have tried to find better ways to monitor what is going on in the blood vessels in tumors and surrounding tissues, especially how to measure the response to drugs.
Now, a team of Memorial Sloan Kettering researchers has shown that a new laser-based technique can generate clear images of tumor blood vessels and reveal in great detail how they change following anti-vascular treatment. The noninvasive imaging method combines the analysis of light and sound to create the picture.
The researchers reported their results on March 12 in Nature Biomedical Engineering.
The team, led by molecular imaging specialist Jan Grimm of the Sloan Kettering Institute and postdoctoral fellow Katja Haedicke, tested the technology in mice given a novel antivascular drug. The technique provided high-resolution images of the blood vessels and how they changed over time after receiving the drug.
“This is the first time somebody has used this method to get an in-depth view of tumor therapies in a living animal,” Dr. Grimm says. “This could be a very valuable research tool for developing cancer drugs — not just antiangiogenesis drugs but other cancer drugs as well.”
A Light Touch
Until recently, the only way to get similar high-resolution live images within tumor tissues has been using intravital microscopy. This requires implanting a small window under the skin to get close to the tumor, which can cause inflammation. “Whenever you do something invasive like this, you have to wonder if the tumor is behaving the way it normally does,” Dr. Grimm says. “Is it giving you true insight into the biological processes?”
The new approach is called raster-scanning optoacoustic mesoscopy (RSOM). It involves sending ultrafast pulses of laser light into tissue. In response, the tissue expands and contracts very quickly. This reaction creates an ultrasound wave that can be measured. Detecting ultrasound bandwidth makes it possible to visualize and distinguish blood vessels of different sizes.
In the study, the researchers used a laser frequency that was ideal for being absorbed by hemoglobin, a protein made by red blood cells. Varying this frequency allowed the team to distinguish oxygen-enriched hemoglobin from oxygen-deprived hemoglobin. This provided an additional dimension to the analysis about what was happening in the tumor blood vessels after therapy. It indicated the blood vessels’ and tumors’ state of health and showed whether the therapy was working.
The scientists demonstrated the potential of this technique by examining how mouse models of cancer responded to a new treatment called vascular-targeted photodynamic therapy (VTP). This treatment, developed by Avigdor Scherz at the Weizmann Institute of Science in Israel, has already been used successfully in people with prostate cancer. It is being further explored at MSK in clinical trials for other cancers.
The researchers used RSOM imaging to study the effects of VTP in mice with various types of cancer implanted under their skin. The researchers were able to get a clear picture of exactly what happened to the tumor blood vessels after treatment. They were able to predict which tumors would respond within a few days of starting therapy.
An Unexpected Resurgence before Collapse
Surprisingly, monitoring a tumor over a longer time period after therapy showed that the blood vessels seemed to respond to VTP in an unexpected way. They initially constricted but then recovered for a brief time. “The vessels look very bad an hour after treatment, then a day later they appear to be back in perfect shape,” Dr. Grimm says. “Then they die off and disappear for good.”
Having visual proof of this short-lived resurgence could help improve therapies, Dr. Grimm explains. Doctors could initially give patients drugs that target the blood vessels as a first strike. Later, they could take advantage of the brief window before the vessels completely collapse.
“When the vessels are flushed with oxygen that last time, you can come with the really hard-core drugs — chemotherapy and targeted therapies,” he says. “So you open the gates and let the bombs in and then everything inside collapses.”
RSOM imaging could also be used to understand how a tumor’s blood vessels react after radiation, Dr. Grimm says.
“This technique lets us see things on a much different scale than anyone could see before,” Dr. Grimm says. “It could be a powerful tool for studying not just antiangiogenesis therapies for cancer but a range of biological processes involving the blood vessels.”